Technological Advancements
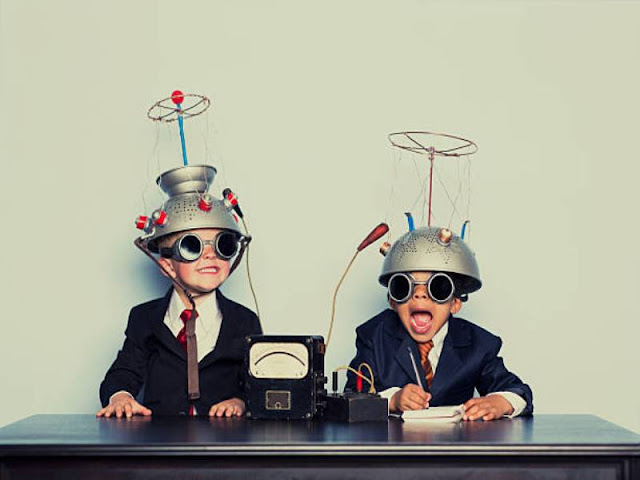
Pioneering the Future of Scientific Discovery
The relentless march of human innovation has consistently pushed the boundaries of knowledge, giving rise to transformative breakthroughs across various fields. Nowhere is this more evident than in the realm of scientific research, where technological advancements have catalyzed revolutionary changes in our approach to understanding the natural world. From high-performance computing and advanced imaging techniques to gene-editing tools like CRISPR-Cas9 and sophisticated simulations, the arsenal of cutting-edge technologies at our disposal has ushered in an era of unprecedented scientific exploration. These tools not only expand our ability to observe and analyze the intricacies of nature but also inspire novel hypotheses and pave the way for groundbreaking discoveries. As we navigate this technologically-driven landscape, we find ourselves on the precipice of a new era of scientific inquiry—one that promises to reshape our understanding of the universe and unlock the secrets of life itself.
At the heart of the technological revolution in scientific
research lies the power of high-performance computing. The exponential
development in computational capabilities over the past few decades has
transformed the way researchers approach complex problems. With the ability to
process vast amounts of data and perform intricate simulations, supercomputers
have become indispensable tools for tackling challenges that were once deemed
insurmountable. For example, computational fluid dynamics simulations have
revolutionized our understanding of fluid behavior in aeronautics and
meteorology, allowing engineers and scientists to design more efficient
aircraft and predict weather patterns with greater accuracy. Additionally,
high-performance computing has played a pivotal role in fields such as
astrophysics, where simulations of cosmic phenomena, such as galaxy formation
and black hole dynamics, provide insights that would otherwise be unattainable
through traditional observation alone.
In the realm of life sciences, advanced imaging techniques
have reshaped our ability to peer into the intricate workings of biological
systems. Techniques like confocal microscopy, electron microscopy, and
super-resolution microscopy have transcended the limitations of conventional
optical microscopy, enabling researchers to visualize cellular structures and
processes at unprecedented resolutions. This has led to breakthroughs in fields
ranging from neuroscience, where neural circuits are being mapped with
unprecedented precision, to cell biology, where the intricacies of cellular
organelles are being unveiled in exquisite detail. Moreover, advanced imaging
has empowered the field of molecular biology, allowing scientists to track the
movements of individual molecules within living cells and elucidate fundamental
processes like DNA replication and protein synthesis.
One of the most groundbreaking technological advancements in
recent memory is the development of CRISPR-Cas9, a revolutionary gene-editing
tool that has transformed genetic research and biotechnology. CRISPR-Cas9's
precision and efficiency in modifying DNA sequences have opened up unparalleled
possibilities for altering genes in various creatures, from bacteria to plants
and animals. The tool's potential to correct genetic defects, treat genetic
diseases, and engineer desirable traits in organisms holds profound
implications for medicine, agriculture, and even environmental conservation.
The accessibility of CRISPR-Cas9 technology has democratized genetic research,
enabling scientists around the world to conduct experiments that were once the
purview of a few specialized laboratories.
Sophisticated simulations stand as another pillar of
technological advancement, offering researchers the ability to recreate complex
phenomena in silico. These simulations are particularly valuable in fields
where real-world experimentation is challenging, dangerous, or
cost-prohibitive. Climate modeling, for instance, leverages simulations to
predict future climate scenarios, aiding policymakers in making informed
decisions about justifying the effects of climate change. Similarly,
simulations have become crucial in drug discovery, enabling researchers to
virtually screen thousands of compounds and predict their interactions with
biological molecules, expediting the drug development process.
The impact of technological advancements in scientific
research is not confined to individual tools; it extends to the convergence of
these tools into multidisciplinary approaches. This convergence amplifies the
power of each technology, facilitating cross-disciplinary insights and
solutions. For instance, the combination of high-performance computing,
advanced imaging, and molecular biology techniques has led to the emergence of
computational biology—a field that integrates computational methods with
biological data to model complex biological systems. Computational biologists
can simulate the behavior of proteins, study protein-ligand interactions, and
predict the folding of biomolecules, thus accelerating drug discovery and
enhancing our understanding of disease mechanisms.
While the potential of these technologies is undeniable,
their utilization also presents challenges and ethical considerations. As
high-performance computing becomes more intricate, the energy consumption
associated with supercomputers raises concerns about sustainability. Balancing
computational power with environmental responsibility becomes imperative. In
the realm of gene editing, the newfound ability to manipulate the genetic code
raises ethical dilemmas, such as the potential for unintended consequences and
the need for responsible use in both research and applications.
Moreover, as simulations grow increasingly complex and
realistic, ensuring the accuracy and reliability of simulation outcomes becomes
a critical concern. The "garbage in, garbage out" principle applies:
if the underlying assumptions or data fed into simulations are flawed, the
results will be equally unreliable. Rigorous validation and verification
processes are essential to maintain the credibility of simulation-based research.
As we stand on the cusp of a technologically-driven era of
scientific discovery, collaboration and interdisciplinary engagement are
essential. Engineers, computer scientists, biologists, physicists, and
researchers from myriad fields must join forces to fully harness the potential
of these technologies. The interdisciplinary approach not only enhances the
development of new tools but also fosters innovative ways of using them.
Cooperative efforts can result in the creation of entirely new research avenues
that transcend traditional disciplinary boundaries.
Institutions and funding agencies play a pivotal role in
facilitating technological advancements in research. They must provide the
necessary resources for acquiring and maintaining cutting-edge equipment, as well
as support for training researchers in utilizing these tools effectively.
Additionally, ethical guidelines and regulatory frameworks must evolve in
tandem with technological progress, ensuring that research is conducted
responsibly and that the benefits of these advancements are accessible to all
of humanity.
In conclusion, technological advancements have irrevocably
altered the landscape of scientific research, providing researchers with an
unprecedented toolkit for exploration. High-performance computing, advanced
imaging techniques, gene-editing tools like CRISPR-Cas9, and sophisticated
simulations have revolutionized the ways we observe, analyze, and manipulate
the natural world. These tools not only empower scientists to delve deeper into
the mysteries of the universe but also pave the way for groundbreaking
discoveries that hold the potential to transform medicine, industry, and our
understanding of life itself. As we move forward, interdisciplinary
collaboration, ethical considerations, and responsible innovation will guide us
toward harnessing the full potential of these technological marvels for the
betterment of humanity.